Fusion energy research has advanced to the point that there is the expectation of fusion energy on the electric grid by 2040. In addition to the substantial continuing government supported developments, there is now a multi-billion dollar commercial energy industry dedicated to developing fusion energy systems. Designing these fusions systems requires simulation codes that account for the full geometric complexity of fusion systems. This has led to the use of unstructured mesh-based methods in fusion energy system simulation codes. As part of the DOE Scientific Discovery through Advanced Computation (SCiDAC) project, SCOREC is providing a range of unstructured mesh technologies, from specialized mesh generators, to massively parallel fusion physics codes, that execute on the DOE’s exascale computers. Efforts have also been initiated to deliver unstructured mesh simulation capabilities to the fusion energy industry.
Primary Contact: Mark Shephard and Jacob Merson
SCOREC Researchers: Merson, Sahni, Shephard, Smith
External collaborators: Princeton Plasma Physics Lab, Lawrence Livermore National Laboratory, Oak Ridge National Laboratory, Argonne National Laboratory, University of Illinois, MIT, Princeton University, General Atomics and Simmetrix Inc.
Developments to Support Modeling of Fusion Energy Systems
Fusion plasma simulation codes developed at PPPL place specific requirements on the unstructured meshes and mesh relationships. To meet their meshing needs specialized mesh generation procedures have been developed that allow fusion energy system analysts to quickly generate well designed meshes meeting the code requirements. The image to the right shows a prototype of a company designed tokamak and a coarse mesh generated that meets the specific needs of the XGC edge plasma simulation code.
In addition to developing specialized mesh generation procedures for fusion plasma simulations, automated mesh generation procedures are being developed to support design optimization workflows for stellarator fusion systems. The images below show a CAD geometry and an automatically generated coarse mesh for blanket and coil design defined in the ParaStell system.
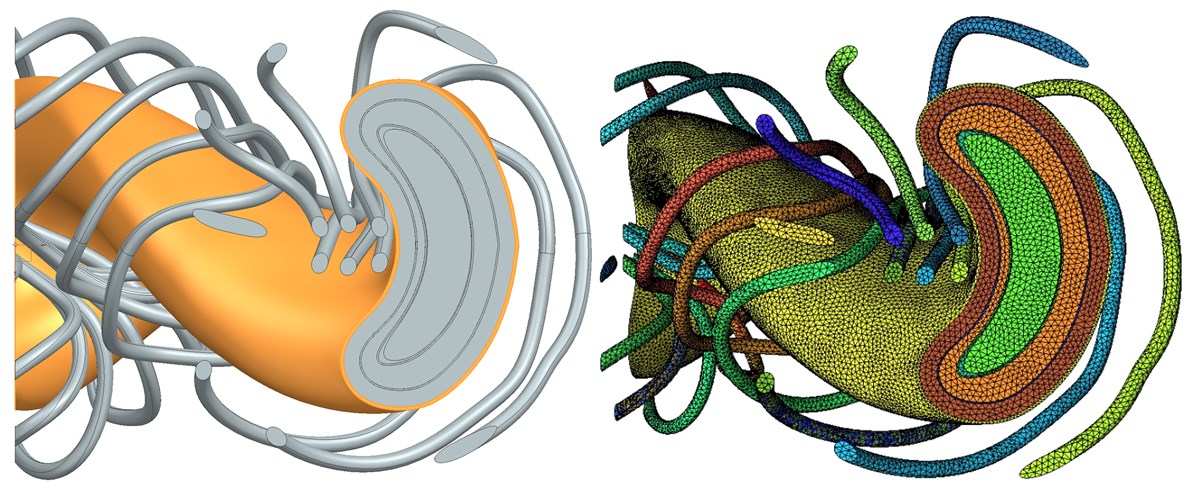
As part of a DOE SciDAC partnership, SCOREC and Simmetrix have been working with MIT, PPPL and LLNL on the development of advanced simulation codes to model the RF heating of fusion plasmas. The MIT, PPPL and LLNL developed PetraM/MFEM high order finite element code provides the base simulation capability needed. Since these simulations require an accurate representation of the antenna geometry, procedures were developed to perform geometry clean-up of the antenna manufacturing CAD models, which are combined tokamak wall geometries and selected physics defined mesh control surfaces to create analysis CAD geometry. To take full advantage of the high-order finite element methods in MFEM, the mesh element must be properly curved to the analysis CAD geometry. To make the most effective use of the computational resources the curved meshes must be adapted to evenly distribute the finite element discretization errors. Curved mesh generation and adaptation procedures have been developed and integrated into the PetraM/MFEM simulation workflow. The images below show a coarse initial curved mesh and a PetraM/MFEM RF result.
In collaboration with ORNL, SCOREC has develop a fully 3D unstructured mesh version of the particle-based global impurity transport code referred to as GITRm. This code supports fully general anisotropic 3D meshes that can account for any level of geometric detail and effectively operate on GPU accelerated exascale computers by employing the PUMIPic mesh/particle library. Additional advances include highly effective methods to support the inclusion of near-wall physics (such as the sheath electric field) and an effective procedure to support multi-species impurity simulations. The images show the mesh for a tokamak example that involves V-shaped small-angle slot (SAS-V) divertor and small probes located far from the divertor, where a graded mesh was used to support the accurate modeling of the interactions of the impurity particles with the SAS-V divertor and probes. ORNL and General Atomics are using GITRm to perform impurity physics studies involving complex geometries.
The M3D-C1 core fusion plasma fusion code developed at the Princeton Plasma Physics Laboratory employs a high order C1 finite element to solve the extended MHD equations. M3D-C1 employs the PUMI mesh infrastructure including its mesh adaptation capabilities. The image shown compares the meshes and solution results for three time steps of a pellet injection simulation using a uniform mesh and and an adaptively refined mesh.
In collaboration with ORNL and other SciDAC partners, SCOREC has deployed coupling technologies to support coupling of gyrokinetic microturbulence (XGC,GTC), neutral beam injection (Beam 3D, GNET), neutral particle recycling (DEGAS2), and Magnetohydrodynamics (BSTING). RPI technologies have also been incorporated into Fusion-IO.
Key Publications:
- Nath, D.D., Srinivasaragavan, V.V., Younkin, T.R., Diamond, G., Smith, C.W., Hayes, A., Shephard, M.S. and Sahni, O., 2023. A 3D unstructured mesh based particle tracking code for impurity transport simulation in fusion tokamaks. Computer Physics Communications, 292, p.108861. 10.1016/j.cpc.2023.108861
- Meredith, L.T., Rezazadeh, M., Huq, M.F., Drobny, J., Srinivasaragavan, V.V., Sahni, O. and Curreli, D., 2023. hPIC2: A hardware-accelerated, hybrid particle-in-cell code for dynamic plasma-material interactions. Computer Physics Communications, 283, p.108569. https://doi.org/10.1016/j.cpc.2022.108569
- Riaz, U., Seol, E.S., Hager, R. and Shephard, M.S., 2024. Modeling and meshing for tokamak edge plasma simulations. Computer Physics Communications, 295, p.108982. https://doi.org/10.1016/j.cpc.2023.108982
- Nath, D.D., Younkin, T.R., Guterl, J., Shephard, M.S. and Sahni, O., 2025. A GPU‐Accelerated 3D Unstructured Mesh Based Particle Tracking Code for Multi‐Species Impurity Transport Simulation in Fusion Tokamaks. Contributions to Plasma Physics, p.e202400073. doi.org/10.1002/ctpp.202400073
- Zhang, C., Diamond, G., Smith, C.W. and Shephard, M.S., 2023. Development of an unstructured mesh gyrokinetic particle-in-cell code for exascale fusion plasma simulations on GPUs. Computer Physics Communications, 291, p.108824. https://doi.org/10.1016/j.cpc.2023.108824
- Shephard, M.S., Merson, J., Sahni, O., Castillo, A.E., Joshi, A.Y., Nath, D.D., Riaz, U., Seol, E.S., Smith, C.W., Zhang, C. and Beall, M.W., 2024. Unstructured mesh tools for magnetically confined fusion system simulations. Engineering with Computers, 40(5), pp.3319-3336. doi.org/10.1007/s00366-024-01976-2
- Siboni, M.H. and Shephard, M.S., 2022. Adaptive workflow for simulation of RF heaters. Computer Physics Communications, 279, p.108434. doi.org/10.1016/j.cpc.2022.108434
Software:
- STOMMS
- GITRm
- XGCm